Nobel Prize in Physiology or Medicine 2024: Victor Ambros and Gary Ruvkin (microRNAs)
for the discovery of microRNA and its role in post-translational gene regulation
The Nobel Prize committee just announced the winners of the Physiology/Medicine award in 2024, and the award goes to Victor Ambros and Gary Ruvkun for the discovery of microRNA and its role in post-translational gene regulation.
The content of this post is edited from the original at https://www.nobelprize.org/prizes/medicine/2024/press-release/
The information stored within our chromosomes can be likened to an instruction manual for all cells in our body. Every cell contains the same chromosomes, so every cell contains exactly the same set of genes and exactly the same set of instructions. Yet, different cell types, such as muscle and nerve cells, have very distinct characteristics. How do these differences arise? The answer lies in gene regulation, which allows each cell to select only the relevant instructions. This ensures that only the correct set of genes is active in each cell type.
This differentiation of cells into the different tissues and organs in our body starts from the first cell of the individual: indeed, that original single cell, with a nucleus containing the DNA that we inherited from our mum and dad, contains the “list of parts” of all the genes that, when expressed at the right time and the right place, in a carefully choreograph developmental process, will turn that single cell into the full body of the animal that they encode for. The entire code for the “list of parts” is present in each cell, but each cell knows what step in the developmental process needs to act upon based on the genomic code and the state of the cell, including the expression of the microRNAs which will help direct this process.
Victor Ambros and Gary Ruvkun were interested in how different cell types develop. They discovered microRNA, a new class of tiny RNA molecules that play a crucial role in gene regulation. Their groundbreaking discovery revealed a completely new principle of gene regulation that turned out to be essential for multicellular organisms, including humans. It is now known that the human genome codes for over one thousand microRNAs. Their surprising discovery revealed an entirely new dimension to gene regulation. MicroRNAs are proving to be fundamentally important for how organisms develop and function.
These 1,000-odd microRNAs are a group of molecules that is found in humans, as well as the majority of mammalian and vertebrate species, which means not only human have them, but also the ancestors which we came from. This also means that the human microRNAs relate to the mouse microRNAs, those in dogs, cats, cows, horses, elephants, etc.
One of the best-studied microRNAs in humans is miR-21. It has been extensively researched due to its involvement in numerous biological processes, particularly in cancer biology. miR-21 is often referred to as an "oncomiR" because of its role in promoting oncogenesis, including the regulation of genes involved in cell proliferation, apoptosis (programmed cell death), and invasion.
Key areas of miR-21 research:
Cancer: It is overexpressed in many types of cancers, including breast, lung, liver, colorectal, and prostate cancer. Its targets include tumor suppressor genes such as PTEN and PDCD4.
Cardiovascular diseases: miR-21 has also been studied in relation to heart diseases, where it plays a role in cardiac hypertrophy, fibrosis, and heart failure.
Inflammatory diseases: It has been linked to immune regulation, particularly in inflammatory diseases like rheumatoid arthritis.
Other well-studied microRNAs include let-7, which is involved in development and tumor suppression, and miR-155, which plays a role in immune response and inflammation.
We can see hop miR-21 is present in humans and all mammalian species, as well as bony fishes, and even the Coelacanth, an ancestral type of species with which we are connected in our evolutionary history.
The picture below shows this evolutionary relationship for the RNA sequence of miR-21:
https://useast.ensembl.org/Homo_sapiens/Gene/Compara_Tree?collapse=none;db=core;g=ENSG00000284190;r=17:59841266-59841337;t=ENST00000362134
Essential regulation
This year’s Nobel Prize focuses on the discovery of a vital regulatory mechanism used in cells to control gene activity. Genetic information flows from DNA to messenger RNA (mRNA), via a process called transcription, and then on to the cellular machinery for protein production. There, mRNAs are translated so that proteins are made according to the genetic instructions stored in DNA. Since the mid-20th century, several of the most fundamental scientific discoveries have explained how these processes work.
Our organs and tissues consist of many different cell types, all with identical genetic information stored in their DNA. However, these different cells express unique sets of proteins. How is this possible? The answer lies in the precise regulation of gene activity so that only the correct set of genes is active in each specific cell type. This enables, for example, muscle cells, intestinal cells, and different types of nerve cells to perform their specialized functions. In addition, gene activity must be continually fine-tuned to adapt cellular functions to changing conditions in our bodies and environment. If gene regulation goes awry, it can lead to serious diseases such as cancer, diabetes, or autoimmunity. Therefore, understanding the regulation of gene activity has been an important goal for many decades.
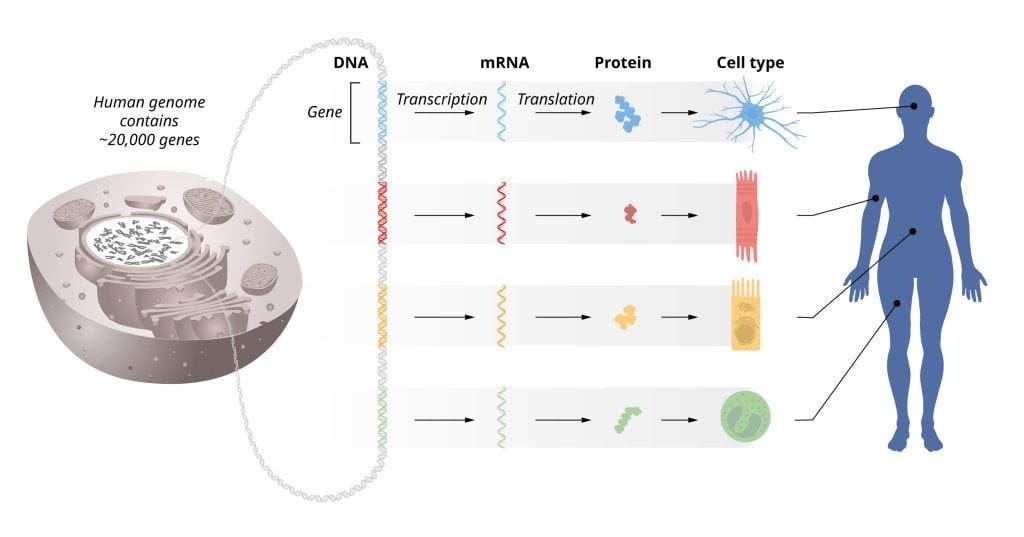
In the 1960s, it was shown that specialized proteins, known as transcription factors, can bind to specific regions in DNA and control the flow of genetic information by determining which mRNAs are produced. Since then, thousands of transcription factors have been identified, and for a long time it was believed that the main principles of gene regulation had been solved. However, in 1993, this year’s Nobel laureates published unexpected findings describing a new level of gene regulation, which turned out to be highly significant and conserved throughout evolution.
Research on a small worm leads to a big breakthrough
In the late 1980s, Victor Ambros and Gary Ruvkun were postdoctoral fellows in the laboratory of Robert Horvitz, who was awarded the Nobel Prize in 2002, alongside Sydney Brenner and John Sulston. The later were working in Cambridge (UK), and were part of a highly productive group of scientists working at the MRC-LMB.
The new MRC LMB labs, with it’s chromosome-shaped building, were inaugurated about a decade ago, and have since then seen Astrazeneca join them as neighbors in their new doughnut-shaped headquarters at the Addenbrooke’s Biotech campus.
In Horvitz’s laboratory, they studied a relatively unassuming 1 mm long roundworm, C. elegans. Despite its small size, C. elegans possesses many specialized cell types such as nerve and muscle cells also found in larger, more complex animals, making it a useful model for investigating how tissues develop and mature in multicellular organisms. Ambros and Ruvkun were interested in genes that control the timing of activation of different genetic programs, ensuring that various cell types develop at the right time. They studied two mutant strains of worms, lin-4 and lin-14, that displayed defects in the timing of activation of genetic programs during development. The laureates wanted to identify the mutated genes and understand their function. Ambros had previously shown that the lin-4 gene appeared to be a negative regulator of the lin-14 gene. However, how the lin-14 activity was blocked was unknown. Ambros and Ruvkun were intrigued by these mutants and their potential relationship and set out to resolve these mysteries.
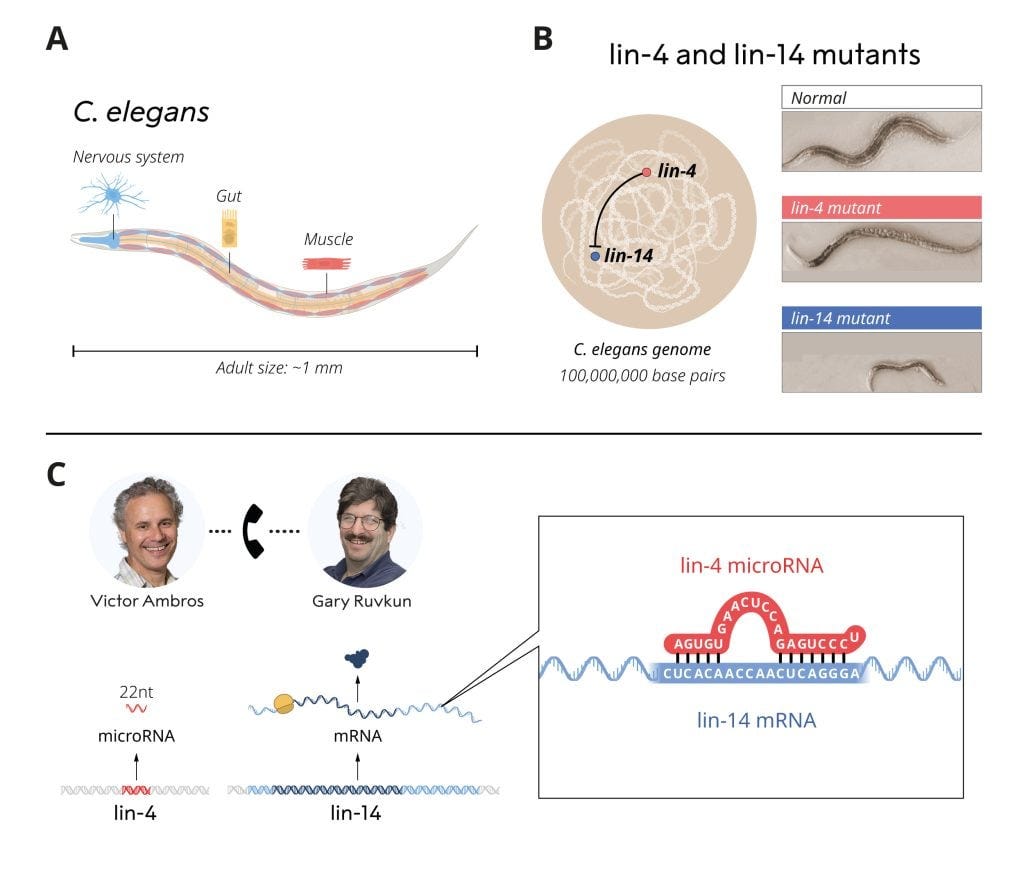
After his postdoctoral research, Victor Ambros analyzed the lin-4 mutant in his newly established laboratory at Harvard University. Methodical mapping allowed the cloning of the gene and led to an unexpected finding. The lin-4 gene produced an unusually short RNA molecule that lacked a code for protein production. These surprising results suggested that this small RNA from lin-4 was responsible for inhibiting lin-14. How might this work?
Concurrently, Gary Ruvkun investigated the regulation of the lin-14 gene in his newly established laboratory at Massachusetts General Hospital and Harvard Medical School.
John Harvard, for whom Harvard University is named, was a graduate of Emmanuel College, Cambridge. He attended Emmanuel College, receiving his B.A. in 1632 and M.A. in 1635. After emigrating to the Massachusetts Bay Colony, Harvard bequeathed half of his estate and his library to the institution that would become Harvard University.
There is a John Harvard statue with is a mainstay of Harvard Yard. But as we know from the movie The Social Network, the statue is not all that it seems. It’s actually nicknamed the “statue of three lies” because of all the inaccuracies inscribed on it.
The statue isn’t of John Harvard. // 2. John Harvard wasn’t the founder of Harvard University, and // 3. Harvard wasn’t founded in 1638.
https://summer.harvard.edu/blog/the-3-lies-of-harvard/
Back to microRNAs…
Unlike how gene regulation was then known to function, Ruvkun showed that it is not the production of mRNA from lin-14 that is inhibited by lin-4. The regulation appeared to occur at a later stage in the process of gene expression, through the shutdown of protein production. Experiments also revealed a segment in lin-14 mRNA that was necessary for its inhibition by lin-4. The two laureates compared their findings, which resulted in a breakthrough discovery. The short lin-4 sequence matched complementary sequences in the critical segment of the lin-14 mRNA. Ambros and Ruvkun performed further experiments showing that the lin-4 microRNA turns off lin-14 by binding to the complementary sequences in its mRNA, blocking the production of lin-14 protein. A new principle of gene regulation, mediated by a previously unknown type of RNA, microRNA, had been discovered! The results were published in 1993 in two articles in the journal Cell.
The published results were initially met with almost deafening silence from the scientific community. Although the results were interesting, the unusual mechanism of gene regulation was considered a peculiarity of C. elegans, likely irrelevant to humans and other more complex animals. That perception changed in 2000 when Ruvkun’s research group published their discovery of another microRNA, encoded by the let-7 gene. Unlike lin-4, the let-7 gene was highly conserved and present throughout the animal kingdom. The article sparked great interest, and over the following years, hundreds of different microRNAs were identified. Today, we know that there are more than a thousand genes for different microRNAs in humans, and that gene regulation by microRNA is universal among multicellular organisms.
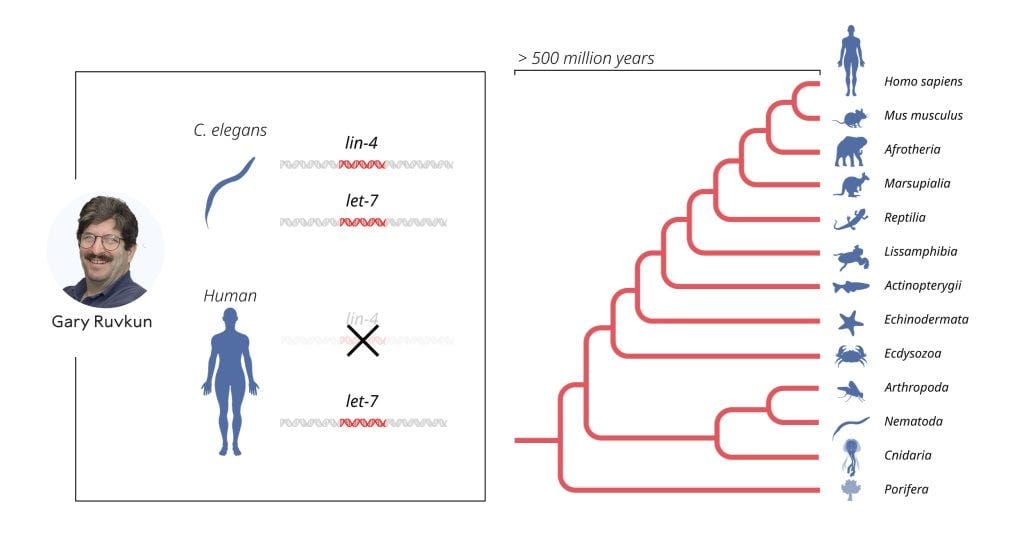
In addition to the mapping of new microRNAs, experiments by several research groups elucidated the mechanisms of how microRNAs are produced and delivered to complementary target sequences in regulated mRNAs. The binding of microRNA leads to inhibition of protein synthesis or to mRNA degradation. Intriguingly, a single microRNA can regulate the expression of many different genes, and conversely, a single gene can be regulated by multiple microRNAs, thereby coordinating and fine-tuning entire networks of genes.
Cellular machinery for producing functional microRNAs is also employed to produce other small RNA molecules in both plants and animals, for example as a means of protecting plants against virus infections. Andrew Z. Fire and Craig C. Mello, awarded the Nobel Prize in 2006, described RNA interference, where specific mRNA-molecules are inactivated by adding double-stranded RNA to cells.
Tiny RNAs with profound physiological importance
Gene regulation by microRNA, first revealed by Ambros and Ruvkun, has been at work for hundreds of millions of years. This mechanism has enabled the evolution of increasingly complex organisms. We know from genetic research that cells and tissues do not develop normally without microRNAs. Abnormal regulation by microRNA can contribute to cancer, and mutations in genes coding for microRNAs have been found in humans, causing conditions such as congenital hearing loss, eye and skeletal disorders. Mutations in one of the proteins required for microRNA production result in the DICER1 syndrome, a rare but severe syndrome linked to cancer in various organs and tissues.
Ambros and Ruvkun’s seminal discovery in the small worm C. elegans was unexpected, and revealed a new dimension to gene regulation, essential for all complex life forms.
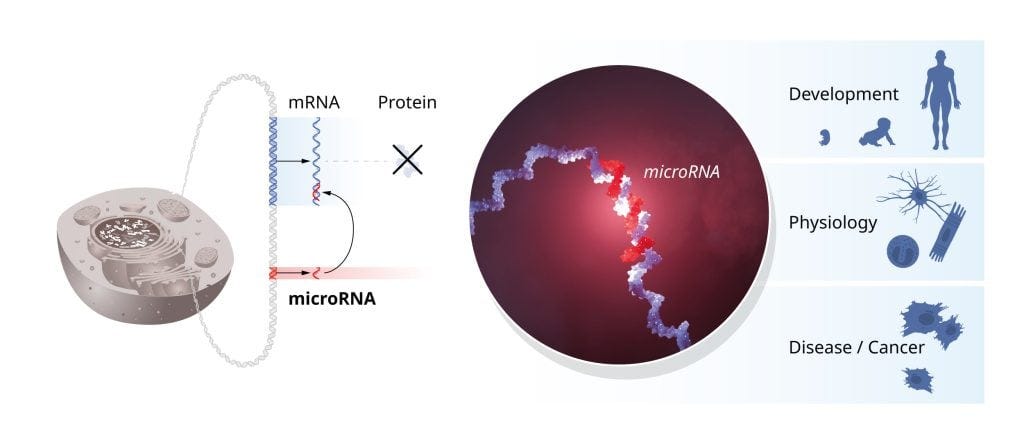
Key publications
Lee RC, Feinbaum RL, Ambros V. The C. elegans heterochronic gene lin-4 encodes small RNAs with antisense complementarity to lin-14. Cell. 1993;75(5):843-854. doi:10.1016/0092-8674(93)90529-y
Wightman B, Ha I, Ruvkun G. Posttranscriptional regulation of the heterochronic gene lin-14 by lin-4 mediates temporal pattern formation in C. elegans. Cell. 1993;75(5):855-862. doi:10.1016/0092-8674(93)90530-4
Pasquinelli AE, Reinhart BJ, Slack F, Martindale MQ, Kurodak MI, Maller B, Hayward DC, Ball EE, Degnan B, Müller P, Spring J, Srinvasan A, Fishman M, Finnerty J, Corbo J, Levine M, Leahy P, Davidson E, Ruvkun G. Conservation of the sequence and temporal expression of let-7 heterochronic regulatory RNA. Nature. 2000;408(6808):86-89. doi:10.1038/35040556